Today, hydrogen is highly praised by politicians as the energy of the future. Indeed, hydrogen is not a primary energy but a vector of energy. However, the hydrogen of the future could be produced from decarbonized electricity and then allow the decarbonization of many chemical or energy uses (production of liquid fuels or fertilizers, mobility) and replace natural gas directly or after conversion into methane. With such potential, Is hydrogen the Swiss army knife for energy transition?
NucAdvisor has delved deeper into one aspect of this broad question: "power-to-power" (P-to-P), that is, the decarbonization of electricity generation by introducing large amounts of intermittent electrical energy (wind and solar) into the electrical mix. The name of the game is to produce hydrogen by electrolysis when intermittent electricity exceeds demand, and to use fuel cells to convert this hydrogen into electricity when intermittent production is insufficient. The concept at first glance is attractive: the surplus electricity would be free and P-to-P chain is clean (no fine particles, no greenhouse gases). But what investment will be required, and what will be the cost of the electricity generated?
NucAdvisor has developed and applied to the French situation a simplified model with the following assumptions:
Demand for electricity remains constant in the future, and there are no exports (not to increase production needs) nor imports (neighboring countries will reserve their decarbonized electricity)
Nuclear, coal and gas electricity generation are phased out
Hydropower and biomass production are stable: no hydroelectric sites are available in France and biomass is reserved for heat or biomethane production
On the other hand, the production of solar and wind energy is hugely increased
Chemical batteries smooth daily production, electrolyzers provide hydrogen in case of excess of electricity generation; stationery fuel cells convert hydrogen into electricity to power the grid in case of insufficient supply
Meteorology (solar and wind resources) is that of a reference year; to account for intra- and interannual variability, four different years (2015 to 2018) were taken as a baseline year.
The model ensures compliance with two key constraints: 1) the supply-demand balance must be ensured at all times; 2) hydrogen stock should always be positive.
The parameters calculated by the model are 1) the required power of renewable energy; 2) the required power of electrolyzers and fuel cells. Based on these elements, the average cost of electricity generated is calculated. A short summary of the main results is presented, corresponding to an average case.
Table 1 is a summary of the renewable capacities needed to replace 63 GW of nuclear installed capacities presently generating ~415 TWh/y (plus ~38 TWh generated by coal and gas-powered plants). This mix takes into account the available potential of different sources of renewable energies. Other mixes (respective shares of offshore, onshore and solar) would be possible, but orders of magnitude would remain the same, and more constraints due to the footprint of renewable energies, and their limited potential, would have to be faced.

It turns out that 1 GW of nuclear energy has to be replaced with ~3,5 GW of wind and solar. It is the combined result of the relatively low load factor of renewables (29%) compared to nuclear (~80%) and of the loss of energy in the P-to-P process (Efficiency of electrolysis and fuel cells : 64% and 43% respectively, i.e. 27,5 % for the full chain).
When averaging the results on the basis of reference years 2015 to 2018, the key parameters of the electrical mix can be derived. There orders of magnitude are summarized in Table 2.
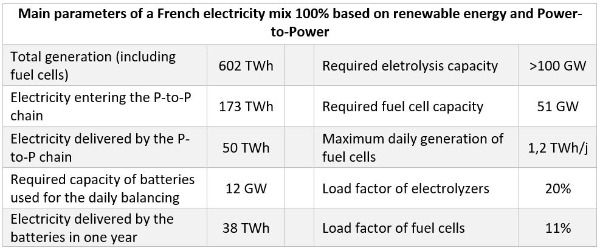
Figure 1 graphically shows the generation-consumption balance for an average reference year.
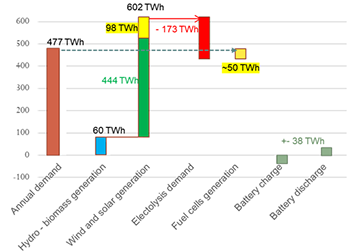
To satisfy a final consumption of 475 TWh of electricity, more than 600 TWh must be produced to compensate for losses in the Power-to-Power chain. In addition to the wind and solar production resources (more than 540 GW), one hundred GW of electrolyzers and more than 50 GW of fuel cells must be added; all to replace about 60 GW of nuclear energy.
Such an electrical system poses obvious feasibility problems. With the technologies available today, we do not know how to ensure the frequency balance of a network that would have very few rotating machines delivering alternating current. But other rather insurmountable difficulties would also arise:
The required photovoltaic energy capacity (in the order of 75 GW (generating ~98 TWh) exceeds the French production potential (estimated at 65 GW), except to use land currently devoted to food production.
The spatial footprint of electrolyzers and fuel cells would be considerable. Indeed, their power density is quite low, and they require many auxiliary equipment. More than 5% of the national territory is expected to be devoted to electricity generation and the P-to-P chain.
Hydrogen storage would require the construction of approximately 200 saline cavities of 500,000 m3 capacity. Presently, there are ~10 such cavities to store natural gas. Beyond social acceptance, the geology of the French territory would not allow such an extension, and neighboring countries would have to agree to realize these capacities on their territory for the French benefit.
The order of magnitude of the production cost of such an electrical mix, replacing 415 TWh of nuclear electricity, can be estimated. Detailed assumptions are given in the reference document. It is assumed that the cost of producing solar and wind energy would be in the order of 60 euros/MWh, and the CAPEX of electrolyzers and fuel cells would decrease to around 1,000 euros/kW, a significant improvement over current cost.
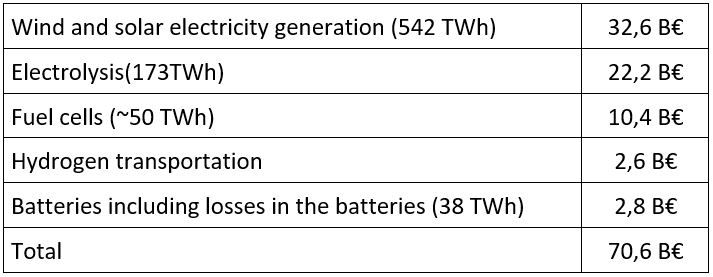
In total, the cost of the 415 TWh presently generated by nuclear plants would amount to 170 euros/MWh compared to 80 euros/MWh for the production of "new nuclear" (Gen. 3) if standardized units were built: the direct generation cost is multiplied by 2,5. The main reason for this result is the very low load factor of electrolyzers and fuel cells (20% and 10% respectively) Which would make such system uneconomic. These factors are a direct consequence of the intermittency of wind and solar electricity: even if the model does not size the electrolyzers for maximum power and accepts to lose a fraction of the excess production, electrolyzers must be sized at a level well above the average power at which they will be used. As for fuel cells, the sizing of the capacity to be installed is constrained: demand must be met even when wind and solar power production is very low. And therefore, a fuel cell capacity high enough to satisfy the peak is needed, even if rarely used.
Are these results based on unfavorable assumptions? Unfortunately, no, the main assumptions are all conservative. The main hypothesis of a stable electricity consumption is not really plausible. Indeed, the phase-out of carbon energies (coal, oil and gas) if one aims for a "carbon neutral" economy and even by making considerable savings will require more electricity used directly in industry, mobility, or heating, or used to produce hydrogen or methane. The French forecast is that electricity demand will exceed 600 TWh in the 2050s compared to 475 TWh taken into account in our scenario. Other conservative hypotheses have been made in this scenario, such as: the cost of storing hydrogen being identical (per m3) to the cost of storing natural gas; no loss in hydrogen transport and storage; no loss in the transmission of electricity in the P-to-P system, etc. Even if each assumption of a medium-term scenario is questionable, and in view of the orders of magnitude, results prove that exiting nuclear power will undoubtedly have an incredibly significant cost.
These conclusions apply to all countries having resources similar to France: average solar and wind potential and limited additional water resources. In such a context, intermittent energies, even with energy storage, cannot ensure the decarbonization of electricity generation without significant additional costs. The alternative envisaged by Germany, Korea, Japan, etc. relies on massive imports of hydrogen from countries well-endowed with solar and wind. The P-to-P strategy is a bet on the construction of a competitive supply chain. Would this bet be lost, these countries will emit CO2, or import nuclear electricity from their wise neighbors relying on nuclear power?
Comments